Multiphoton (Fluorescence) Microscopy
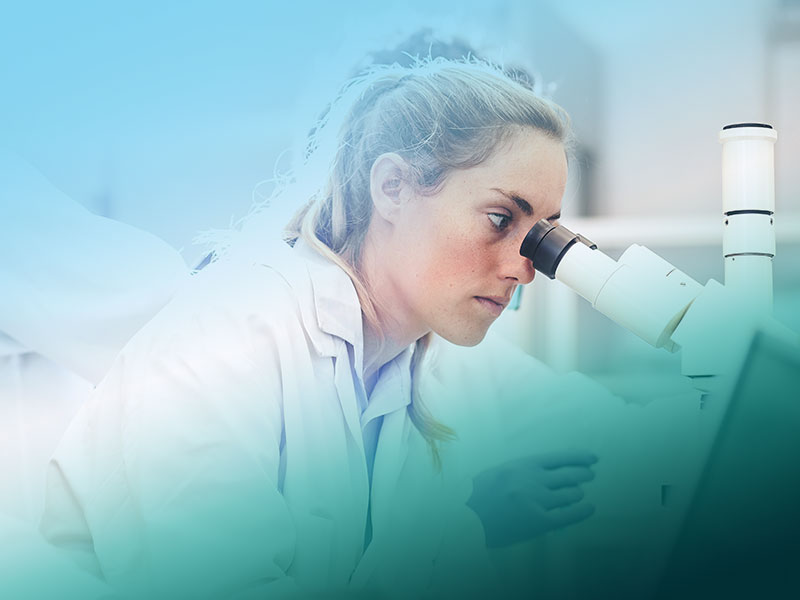
In multiphoton microscopy, molecules are transformed into an electronically excited state by several photons. From this state, these molecules relax back into the electronic ground state by emission of a single photon. In consequence, the emitted photon has a higher energy (shorter wavelength) than the photons for excitation.
In order to excite molecules by several (long-wave) photons, extreme power densities of light are required, since the photons have to interact with the molecule quasi simultaneously. In two-photon excitation, the interaction is explained quantum mechanically in such a way that the molecule is in a raised virtual energy level by a first photon as long as the photon interacts with the molecule (few femtoseconds, 10-15 sec).
If within this short time a second photon comes and interacts with the molecule, it can be transformed into a now real, electronically excited state. From this level, the molecule relaxes into the ground state. This can work in different ways: Either it is a non-fluorescent molecule – in this case, a photon with double the energy is 'simply' emitted. For example, if one irradiates the sample with photons that have a wavelength of 800 nm, one would expect an emission at 400 nm. This effect is also called 'Second Harmonic Generation' (SHG), which is also used in many laser crystals, e.g. to generate laser light of the wavelength 532 nm, which originally comes from a NdYAG laser emitting at 1064 nm.
Vibration Excited State in Fluorescence
If it is a fluorescent molecule, the molecule is not only lifted by the second photon into an electronically excited state, but also into a vibrationally excited state, from which it first relaxes radiation-free into the electronically excited, but vibration ground state. From this state, it changes to the electronically ground state but vibrationally excited state by emitting a fluorescence photon (see article » Fluorescence Microscopy). Besides this, the excitation can also be performed by three, four or even more photons, but this requires ever higher power densities of the light for the excitation, since the time span for the excitation does not increase further.
As for the 'normal' one-photon excitation spectra of molecules, spectra for multiphoton excitation can be measured. Since the effect of multiphoton excitation was predicted by Nobel Prize winner Maria Goeppert-Mayer in her dissertation 1931, the unit of the cross section of molecules for two-photon excitation is measured in 'Goeppert-Mayer' (GM) in her honor.
Advantages and Disadvantages of Multiphoton Microscopy
Multiphoton microscopy has several advantages and disadvantages compared to single-photon microscopy:
Seeing Insights in Deeper Layers
Due to the reasing that the excitation wavelengths are mostly in the infrared region, the excitation light is scattered less in (biological / organic) samples. This allows to penetrate into deeper layers of the sample and to scan a larger volume with the microscope without having to much problems due to scatteirng and a large deformation of the excitation PSF.
Fading Of The Sample
Since multiphoton excitation can only be achieved by very high power densities, this is only possible by strongly focused beams. This leads to the fact that, comparable to confocal microscopy, one has to scan the sample point by point, which makes imaging rather slow. In comparison to confocal microscopy, however, no pinhole is needed before detection, since the emission can only take place in the focus of the excitation anyway, which tends to detect more light. The emission scales with the square of the excitation power (for two-photon excitation). Due to the high power densities in the focus, the samples usually bleach relatively fast, which is probably the biggest disadvantage of multiphoton microscopy, besides the expensive lasers.
Resolution
On one hand, the resolution of multiphoton microscopy is better by the root of the number of required photons for excitation. I.e. increased by root 2 for two photons. However, it should be noted, that the photons have (usually) a wavelength twice as long and thus intrinsically offer only half as good resolution as the photons that would be needed for single-photon excitation. Therefore the resolution is effectively worse by the root of the number of required photons compared to the equivalent single-photon excitation.
Reflection of Emissions
As in classical fluorescence microscopy, in multiphoton microscopy the emission is collected using the same objective (epi-fluorescence setup). Since the emission photons have a shorter wavelength than the excitation photons, one has to make sure that the emission is reflected by a suitable long-pass dichroic and not transmitted as in classical single-photon fluorescence microscopy.
Dispersion
In order to be able to achieve the power densities required for multiphoton microscopy at all, pulsed lasers with very short pulse lengths must be used (usually around 50 fs to 300 fs long pulses, rarely more than 1-2 ps). However, this also means that the laser pulses react critically to dispersion, e.g. when they pass through glass, which increases the pulse length and reduces the peak intensity. Therefore, a pulse compressor is usually needed for the laser pulses in the excitation path to compensate this linear dispersion.
Dielectric filters
Dielectric filters can cause big problems concerning the dispersion. The problem rises by the reflections on dielectric filters. In these filters the light is reflected at many different interfaces of the dielectric coating in different depths of the coating. This results in a nonlinear dispersion of the rays, which cannot be compensated by compression. One must pay attention to the "Group Delay Dispersion" (GDD) of the beam splitters. In transmission, the GDD of the beam splitters is quasi linear and depends on the thickness of the substrate. This can be compensated. In reflection, the different portions of the short-pulsed laser light (not monochromatic!) penetrate the substrate to different depths and in a non-linear manner, so that each portion of the light travels a different path length.
However, it is possible to minimize the GDD for a certain wavelength range when designing multiphoton beamsplitters. Special 'Low Dispersion Dichroics' are developed for this purpose.
Matching Beam Splitters and Optical Filters
At AHF analysentechnik you will find a large selection of suitable beam splitters, which have proven themselves in a wide range of multiphoton microscopy applications. We would be pleased to advise you in selecting the appropriate filters for your microscope and your specific application. You will benefit from our many years of interdisciplinary experience in microscopy.